Jay Dean, PhD, is a professor of molecular pharmacology and physiology at the University of South Florida (USF), where he studies the effects of oxygen, carbon dioxide, and barometric pressure on the mammalian central nervous system. His research has increased our understanding of oxygen toxicity seizures and the warning signs and symptoms before a seizure. Dean and his colleagues have continued to work toward preventing oxygen toxicity via ketosis and administering supplements.
What is your academic background?
I did my undergraduate work in biology at Central Michigan University and received my master’s degree in biological sciences at Michigan Technological University. During my master’s program, I worked on comparative respiratory control with northern water snakes, which are pugnacious 3-foot-long snakes that like to bite you. I studied the effects of temperature and carbon dioxide rebreathing on their ventilation and blood pH regulation.
After that, I went to Ohio State University for my doctorate to continue working in comparative respiratory control but instead ended up at a lab where I did neural-control of body temperature. That’s where I learned about the central nervous system and electrophysiology to study brain cell signaling.
As a postdoc, I got back into respiration, which has always been an interest of mine. At the University of North Carolina at Chapel Hill, I worked on the central carbon-dioxide chemoreceptors, which tell you when you have too much carbon dioxide in your system. That accumulation is your primary stimulus for breathing. I studied carbon-dioxide-sensitive neurons and their location in the mammalian brain stem.
While doing that work, I began to think about other gases and how they affect the brain. I read about how some gases such as nitrogen don’t really affect us at sea level, but putting it in a scuba tank and diving deep enough while breathing it raises concerns about nitrogen narcosis. We also usually do fine with oxygen, but if it gets to be a high enough partial pressure, if you increase the fractional concentration (such as in nitrox), or if you go for pure oxygen, you have to worry about central nervous system oxygen toxicity.
After finishing my doctoral and postdoc work, I got my first faculty position at Wright State and was awarded an in-house seed grant that funded development of my first hyperbaric chamber. I adapted the tools I was using to study the effects of carbon dioxide on brain cells for use under high pressure for oxygen toxicity. That was how I got my foot in the door in undersea medicine. In 2000 I received financial support from the Office of Naval Research Undersea Medicine Program. I came to USF in 2006 and continue to work for ONR Undersea Medicine.
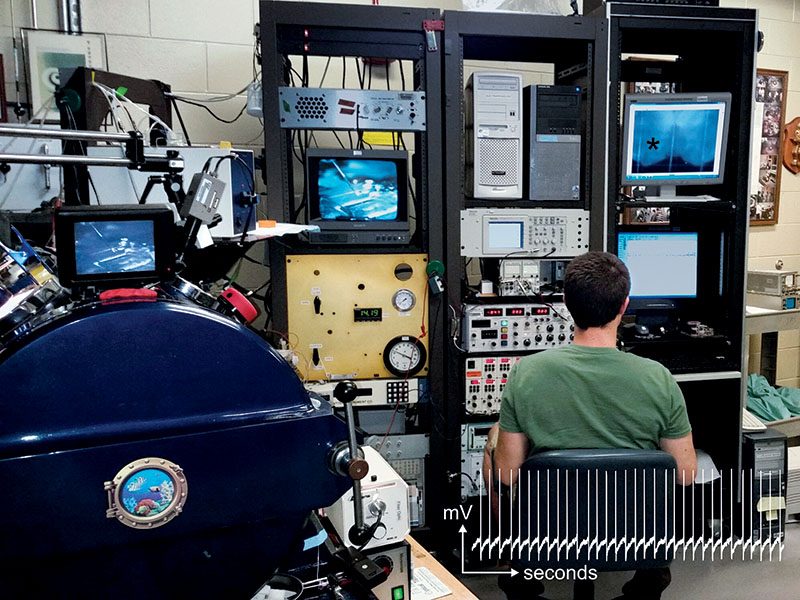
What are you currently working on?
I’m working on the mechanisms and mitigation of central nervous system oxygen toxicity and trying to find the answers to the following questions: Why does the brain suddenly develop seizures when it’s exposed to too much oxygen under pressure? Are there ways to delay it? Are there ways to predict that your level of oxygen exposure is taking you to the point where you’re going to have a seizure? Can we identify physiological markers that will warn us before seizure begins?
The problem is the tremendous variability in sensitivity to hyperbaric oxygen in terms of when seizures occur between individuals and within the same individual from day to day. We don’t quite know why that is.
We asked if a rodent’s breathing would pick up before it had a seizure. Was that possibly an early-warning physiological marker? So we did the experiments and observed that their breathing picked up anywhere from 8 to 15 minutes before a seizure. (Note: The USF Institutional Animal Care and Use Committee (accredited by the Association for Assessment and Accreditation of Laboratory Animal Care International) and the U.S. Department of Defense Bureau of Medicine approves all animal use.)
Since then we’ve looked at other things. We found that there’s an increase in electrodermal resistance, or skin resistance, that precedes the seizure by several minutes. The heart rate tends to slow initially during the dive, and that precedes a seizure by several minutes in an unanesthetized animal. Another marker seems to be a drop in body temperature, although the jury is still out on that. What is clear is that several of these physiologic changes precede and forewarn of impending seizures.
We’ve also successfully delayed seizures in rats by using an exogenous ketone ester developed by my colleague Dominic D’Agostino, PhD. Within 30 minutes their blood profile looked like they had been on the ketogenic diet for a week. Replacing your body’s usual primary energy source (glucose) with ketone bodies seems to have several neuroprotective effects. For example, it decreases free radical production during exposure to hyperbaric oxygen. This process delayed the seizures from 300 percent to 600 percent, creating a longer, safer dive for the rodent. The ketogenic diet will affect the brain’s oxygen consumption and utilization and do several other things that together delay onset of seizures.
Where did your research lead you?
An unanswered, fundamental question that has kept us from targeting the cells critical for seizure genesis is knowing where do oxygen toxicity seizures originate? What parts of the brain are involved? Research indicates that seizures seem to originate at multiple subcortical sites in the brain.
In 2019 we hypothesized that the early, abnormal cardiorespiratory changes that precede seizures suggest that so called “ox-tox trigger zones” exist in cardiorespiratory control centers of the brainstem. These ox-tox trigger zones get stimulated by a big hit of hyperbaric oxygen and begin generating their depolarizing signals. Other ox-tox trigger nuclei start to activate and then amplify and relay the signals. The activation level determines the size and complexity of the seizure. Animal models for oxygen toxicity show that the seizures are quite complex. They can range from subtle to dramatic, depending on how much the brain gets activated.
We’re currently using radiotelemetry to determine parts of brain that are activated during seizure genesis — that is, implanting a transmitter with embedded wires to measure brain activity in various regions and respiratory muscle activity. We’ve adapted the process so we can embed electrodes deep in the brain, where we think the ox-tox trigger nuclei are as well as over the motor cortex, which turns on when the seizure manifests.
Our initial studies show that these brain stem ox-tox trigger zones appear to turn on minutes to tens of minutes before we see seizure activity in the motor cortex or physical convulsions. We can study animals unrestrained, behaving freely, and unanesthetized with these implanted radio telemetry modules. It’s a powerful technique.
Delaying seizures is another current focus. We are using compounds to inhibit nitric oxide synthase, which has been beneficial in delaying seizures. The question is whether that can translate into something the U.S. Food and Drug Administration can approve for use. The holy grail is finding a substance that works in animals and then can be approved for human use.
Why conduct physiological research on rats rather than humans?
Animal research is critical. Everything we know in medicine — including undersea medicine — starts with animal research, particularly with mammals. I’ve always been interested in fundamental questions, plenty of which we could initially answer in animals but not humans. There’s not a lot of basic research in the undersea medical community, and our goal is to have our work translated into larger animals and human divers. Animal research serves an important role in that chain of events, and I’ve been contented to do that for my career.
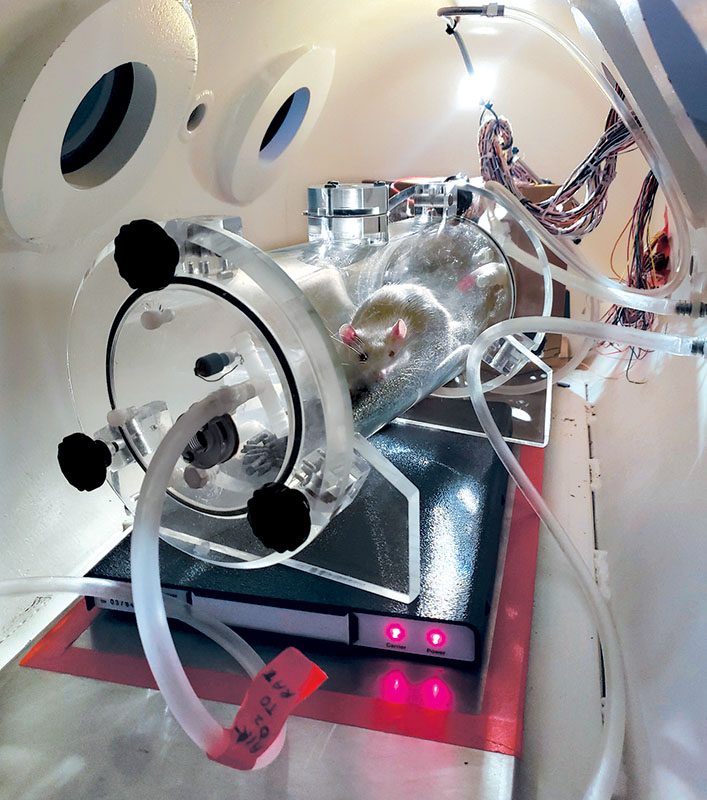
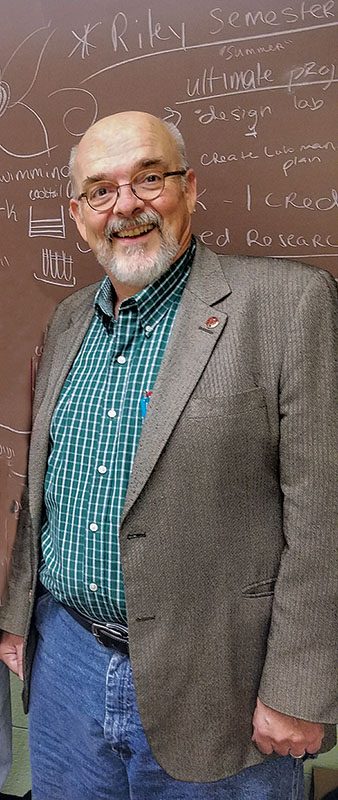
What do you like to do when you’re not in the lab?
My main hobby is studying the history of aviation medicine during World War II. I tell people that I work at depth under hyperbaric pressure and high oxygen during the day, and then I ascend to altitude at night and study hypoxia, decompression, and high altitude.
Fred Hitchcock was a big-time physiologist who ran the aviation medicine lab at Ohio State University during World War II and studied explosive decompression at high altitude. He had just died when I began graduate school at Ohio State. After I passed my comprehensive qualifying exams, I moved into what turned out to be Fred Hitchcock’s office as an emeritus professor. He had no family, so all his stuff was still there. One day I opened a closet to find a huge box filled with films, slides, negatives, and other documents. Later, my first faculty position was at Wright State, which is next door to WPAFB and I started spending Fridays in the archives going through declassified USAAF Aero Medical Lab reports.
An interesting dive-related story from World War II that I uncovered is the development of using aviation oxygen equipment for scuba in a water-ditching emergency. American aviators were drowning before they could safely escape from airplanes ditched at sea during the war. The U.S. Army Air Forces Aero Medical Laboratory at Wright Field tested to see if pilots could use their aviation oxygen mask and walkaround oxygen bottle as scuba equipment to allow for a safe evacuation from their submerged aircraft, and it worked!
I eventually began touring the country to speak about aviation medicine. The aerospace medical community has been excited about the historical perspective. I just gave a talk in Reno, Nevada, at the joint meeting of the Aerospace Medical Association and the Undersea and Hyperbaric Medical Society. I was part of a panel discussion on conquering pressure barrier environments, and I spoke on the research done during World War II.
There’s a lot of overlap in the people who worked in undersea medicine and who continue to work in high-altitude research and vice-versa.
© Alert Diver — Q1 2023