A research physiologist in the biomedical research department at the U.S. Navy Experimental Diving Unit (NEDU) and an assistant professor in the anesthesiology department at Duke University School of Medicine, David J. Doolette, Ph.D., is also an advanced technical and cave diver, an educator and a public speaker. Well published in scientific journals, he has helped make significant advances in the science of diving for more than 20 years.
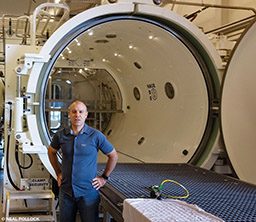
Born and educated in Australia, Doolette did his postdoctoral research on blood flow and the transport of gases, drugs and physiological substances to tissues and organs such as the brain, heart, lungs and muscles. He became an expert in modeling the effects of blood-flow changes and variations in substance-loading parameters, which is ideal for the study of gas transfer during compression and decompression. While researchers with these skills are in high demand in clinical physiology and pharmacology, Doolette made his way to diving physiology.
After he became a diver, Doolette wanted to apply his skills to the science of diving. Decompression illness (DCI) has various forms and degrees of severity, and diagnosis of DCI in field studies based on self-reporting is elusive. Doolette developed a tool to measure health status in divers and calibrated it to diagnose DCI. This tool was later used to study and improve safety for various groups of divers.
He published several studies modeling various aspects of saturation and desaturation before joining the team at the NEDU. Since then he has been in the vanguard of research and development of safe decompression procedures. We appreciate his willingness to tell us more about his work.
How and when did you become a diver?
I have always lived by the sea, and one high school I attended was very close to a rocky coastline, where I would regularly spend my lunch break snorkeling. One day I watched the bubble trails recede from two scuba divers in the water, and I thought, “I want to do that.” In 1979 on the day I turned 16 years old (then the minimum age to learn scuba), I enrolled in a scuba course. I have been involved with diving, for work and play, ever since.
Why did you develop the health survey tool, and what does it do for decompression research?
In the early 1990s a diver-intensive fish-farming industry arose in South Australia. Bluefin tuna were caught at sea and transported to coastal pens, where they were fattened for the sashimi market. At first the industry employed divers with recreational training, and there were a large number of incidents of serious decompression sickness (DCS). I worked with Derek Craig, a diver and health and safety inspector, to characterize the decompression practices. I needed a standardized method for collecting postdive health-outcome information, particularly the symptoms of DCS, from a large number of divers in the field. The ideal method would be having medically trained personnel go into the field to monitor the divers. That was not practical, so I developed and validated the diver health survey, a one-page questionnaire used for self-reporting health status relevant to DCS.
The incidence of DCS in recreational diving is relatively low, and divers are surprised when it occurs because they and their buddies previously dived similar profiles many times without any problem. Could you tell us about variability of decompression outcomes and how that affects your research?
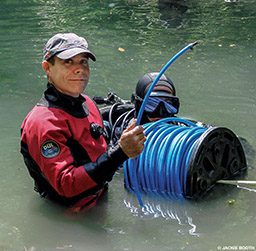
It is because of this question we are having this interview. In 1985 I had finished my undergraduate studies in physiology and was working as a scuba instructor. One day two friends and I were doing a wreck dive; it was a fairly deep air decompression dive, and afterward one of my buddies, who had done thousands of dives previously, developed DCS. That got me thinking, “Why him, and why that day?” and sparked my interest in DCS.
Similar dives may actually be different enough to have a different risk of DCS even if the depth/time profile is the same (which is unlikely outside of research settings). For instance, if divers work hard while at depth or are cold during decompression, their risk of DCS is greatly increased compared to being at rest and warm — as much as if they had doubled their bottom time. Even in a laboratory setting, however, where dives are conducted in a pressurized wet pot where the dive profile, breathing gas, water temperature and work performed can be identical for all practical purposes, we see variability in DCS outcome. In other words, identical dives with the same diver can result in DCS on some occasions and not others.
Since 2000 the Navy has conducted a number of experiments in which an individual dives the identical dive profile numerous times, sometimes resulting in DCS and sometimes not. That is clear evidence that an individual’s susceptibility to DCS changes on a day-to-day basis. There are two important messages for divers: 1) Dives are not completely “safe” or “unsafe” — rather they have a higher or lower risk of DCS; and 2) having done similar dives in the past without incident is not a reason to discount DCS. Modern decompression science is concerned with measuring and predicting DCS risk.
How do dive computers handle the variability of decompression outcomes?
Dive computers and decompression tables handle the person-to-person, day-to-day variability in susceptibility to DCS by providing a choice of relatively conservative decompression guidance, so that most people on most days will be safe if they dive properly.
How should dive computers be evaluated in the development phase?
A dive computer should implement a decompression algorithm that has been subjected to human testing so that the DCS risk within the no-stop limits and decompression schedules is well characterized. The dive computer should then undergo validation testing to make sure it faithfully produces no-stop limits and decompression schedules exactly as intended. This is the approach taken by the U.S. Navy.
What should divers consider when choosing a dive computer?
Unfortunately recreational divers probably do not have many choices of dive computers that implement a decompression algorithm subjected to the same level of human testing as the U.S. Navy dive computer. I am not an expert on recreational dive computers, but I know many implement variants of the ZH-L16 (Bühlmann) decompression algorithm, and the human testing of ZH-L16 is well documented.
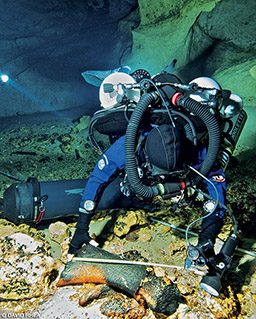
Deep stops have been of interest to the diving public — technical divers in particular. The disputes may not be settled yet, but your team contributed important evidence. Where do we stand regarding the efficacy of deep stops?
We need to consider three types of deep stops. First is the use of deeper than traditional safety stops for recreational no-stop dives, where the total dive time, including the safety stop, is less than accepted no-stop limits. These deep safety stops probably do no harm, but the evidence is conflicting as to whether they are of any benefit compared to traditional safety stops at 10-15 feet of seawater.
Second is the practice that was popular in the early days of technical diving of adding some brief, unscheduled decompression stops deeper than the first prescribed decompression stop and then recalculating (or letting the dive computer recalculate) the additional required decompression time. This will result in a longer total decompression time and, if the stops are not too deep, should be safer than the original schedule, but how much safer has never been rigorously tested.
The third type of deep stop is when a decompression algorithm is designed to redistribute time from shallow decompression stops to deep stops; in other words, compared to a conventional decompression schedule, there are additional deep stops but the total decompression time is the same (or shorter). The theoretical premise is that the deep stops result in fewer and smaller bubbles and so the resulting deep-stops schedule should have lower risk of DCS than a conventional schedule. There is now considerable experimental evidence that these types of deep-stops schedules do not impart a lower risk of DCS than conventional schedules.
How does body temperature affect decompression?
Being very warm on the bottom or being very cold during decompression increases the risk of DCS. Presumably this results from increased blood flow to superficial tissues and therefore faster uptake of inert gas when warm and, conversely, reduced blood flow and slower removal of inert gas when cold. This is probably not of great consequence for divers conducting no-stop dives and certainly not worth divers making themselves deliberately cold on the bottom and risking hypothermia. For divers conducting decompression dives, however, it is worth considering. If a diver becomes very cold during decompression, the time required for decompression is increased. If divers have active heating, such as electrically heated drysuit undergarments, they should use these only enough to stay comfortable while on the bottom and conserve the battery to ensure they can use the heat during decompression.
What should recreational divers do when dive conditions make them exert themselves more than usual?
Work on the bottom increases blood flow and results in faster uptake of inert gas. This will increase the risk of DCS for a no-stop dive. Recreational divers who exert themselves more than usual on the bottom should add some safety by ascending before they reach their no-stop limit.
We now have more ways to study the venous gas bubbles that may occur after diving. What are some of the tools and methods that allow this research?
Venous gas emboli (VGE), the bubbles that occur in the body’s tissues, are transported by venous blood and can be detected in large veins or in the right side of the heart. The number of bubbles is commonly described semiquantitatively (i.e., by a grade on an ordinal scale). Many dives result in detectable VGE but do not result in DCS. VGE after a dive in no way indicates whether the diver will get DCS. In large compilations of experimental dives, however, there is a higher incidence of DCS among dives that resulted in high-grade VGE than in dives that resulted in low-grade VGE. This relationship leads people to use VGE as measure of decompression stress (an index of DCS risk) in dives that do not necessarily result in DCS. This can produce useful information if VGE are the appropriate outcome measure, if the experiment is carefully designed and well executed, and if the results are thoughtfully interpreted.
VGE, however, are not the appropriate outcome measure for all experiments related to decompression. VGE are only meaningful if the experiment is testing an intervention that changes bubble formation and growth in tissue and thereby influences DCS risk. Examples are tests of decompression schedules, diver exertion and diver thermal status. VGE are not an appropriate outcome measure for interventions aimed “downstream” of bubble formation and growth — at the pathophysiological responses to bubbles. An example would be evaluation of methods of treating DCS.
VGE grades following identical dives are quite variable, both between divers and in the same diver on different occasions. Therefore, the VGE grade after a single dive in a single individual is not informative. Only after multiple repetitions of the same dive profile are VGE grades useful. The number of repetitions depends on the research question, but in my opinion there needs to be good justification for less than 20, and 50 would be preferable.
There are two common experimental designs. VGE might be used to validate a decompression table by evaluating selected schedules. Each schedule is dived at least 20 times and “fails” if more than half of the dives results in high-grade VGE; otherwise it “passes.” A more common experimental design, and in my opinion the best use of VGE, is to compare two or more different decompression procedures. Each procedure is dived 50 times, and a significant difference in VGE grades indicates a difference in DCS risk.
There are many technical challenges in executing a good VGE study, but one very important consideration is the frequency and timing of VGE measurements after diving. The only validated index of “decompression stress” is the peak VGE grade measured after diving. This peak might occur any time from immediately after surfacing to several hours later. It is usually practical to measure VGE only periodically, so these measurements need to begin soon after surfacing and continue frequently for two or more hours.
One of many important considerations in interpreting results of VGE experiments is that while a significant difference in VGE grades between two procedures is evidence of a difference in DCS risk, it is not a reliable indicator of how large the difference is. Similarly, for many reasons, failure to find a difference in VGE grades between decompression procedures does not indicate there is no difference in risk of DCS.
© Alert Diver — Q4 Fall 2016