Hyperbaric oxygen therapy (HBOT) is very effective for treating decompression sickness (DCS), but in about one-third of cases it does not provide complete resolution of symptoms. In particular, divers with neurological symptoms who do not receive immediate HBOT respond less well to it later. A challenge in hyperbaric medicine today is to find ways to improve the prognosis for individuals for whom HBOT is ineffective or incompletely effective.
Furthermore, HBOT is not always available; this is true in diving as well as in other settings. Accidents in submarines, high-altitude aviation or space can also cause severe (and perhaps lethal) DCS, and immediate HBOT is not generally available in these situations. The search for therapies that can complement or serve in place of hyperbaric therapy is ongoing.
Accepted adjunct therapies today include surface oxygen, fluid resuscitation, management of plasma glucose, corticosteroids, anticoagulants and management of core temperature. Recent developments with compounds called perfluorocarbons (PFCs) are raising hopes that we may be close to an even more effective adjunct treatment for DCS. The question is how close.
PFCs are synthetic “oils” made up of carbon and fluorine atoms only. They were developed as inert insulating materials in the Manhattan Project during World War II. PFCs are stable and do not react with living tissues but have a large carrying capacity for gases including oxygen, carbon dioxide and nitrogen. The boiling temperatures of various PFCs differ. They can be liquid at room temperature and gas at body temperature.
Uses of PFCs in humans were investigated for many years before they were considered for treating DCS. Compounds with their characteristics had long been sought by pursuers of two major quests in medicine: the quest for liquid breathing and the quest for a blood substitute.
Liquid Breathing
The quest for liquid suitable for breathing began after World War I in the course of research into the treatment of poison-gas inhalation. Early attempts at using saline solutions applied to the lungs of dogs failed. The topic was resurrected after World War II with the introduction of nuclear submarines. The subs operated in very deep water, and if they became disabled at depth their crews could not survive escape. Breathing a liquid medium was studied as a possible way to increase the depth from which crew members could be rescued.
In 1962 J.A. Kylstra and colleagues published the paper “Of Mice as Fish,” an account of a study that showed mammals could breathe a liquid medium. Mice survived immersed in physiological salt solutions and compressed to 160 atmospheres (atm), which is the pressure 1 mile below the surface of the sea. All the animals died of respiratory acidosis because it took great effort to move liquid in and out of lungs, and only minimal ventilation was possible. Thus, for liquid breathing to provide sufficient oxygenation and removal of carbon dioxide, a liquid with a large carrying capacity for these gases was needed. PFCs met these requirements.
Leland C. Clark and Frank Gollan of Birmingham University showed in 1966 that small mammals could survive for an hour completely submerged in PFCs. But further studies demonstrated gas exchange in healthy lungs is impaired in a liquid medium relative to a gas medium. This impairment combined with liquids’ disturbance of normal lung mechanics brought an end to the quest for liquid breathing.
A Blood Substitute
The quest for a substitute for precious blood — always in short supply — was also under way. Supplying oxygen is just one of blood’s many vital functions, but for short-term blood replacement (which could save lives in emergencies) oxygen-carrying capacity is the primary concern. PFCs have been considered for that purpose since the early 1960s. At atmospheric pressure and body temperature, PFCs dissolve large amounts of gases — oxygen and carbon dioxide in particular. Unlike the binding and release of oxygen from hemoglobin in the blood, the dissolution of oxygen in PFCs is entirely passive. When PFC emulsions are administered intravenously, they are loaded with oxygen in the lungs, where the oxygen pressure is high, and release oxygen in tissues where the oxygen tension is low. It is this characteristic of PFCs that is the subject of ongoing research.
A Therapy for DCS
Because of their high nitrogen-carrying capacity it was not long until PFCs caught the attention of diving and hyperbaric physiologists. In the 1980s it was shown that small animals can survive otherwise fatal decompressions if they receive an intravenous emulsion of PFCs immediately after decompressing. Progress in this area proved to be slower than expected, but our knowledge and technologies continue to improve. A definitive answer has not yet been found, but research is ongoing.
How have PFCs been used in medicine?
Steven Hill: PFCs were approved several years ago as oxygen carriers for use as a temporary measure during ischemia in coronary angioplasty procedures. The procedure got so efficient, however, PFCs became unnecessary for angioplasty and stents. The procedure progressed to the point PFCs weren’t needed.
In the late 1990s or early 2000s PFCs advanced into late-stage clinical trials that investigated their ability to enhance hemodilution. Hemodilution is a method of preserving hemoglobin mass during high-blood-loss surgical procedures in people who refuse blood transfusions. PFCs were used to enhance the oxygen-delivery capability of and the amount of dissolved oxygen in the fluid phase of the blood during surgery, which allowed doctors to preserve more of patients’ own red cells for postsurgical readministration. The late-phase trial called for these patients to be significantly hemodiluted, but we ran into problems with the loss of other elements of blood such as viscosity and clotting factors. There was no problem with oxygenation or oxygen delivery — the PFCs did what they were supposed to do. But the patients became hypotensive and required clotting factors and platelets at the end of the procedure because they developed acquired coagulopathies.
It’s important to think of PFCs as a temporizing measure. You can’t give PFCs over a long period because the body needs time to get rid of them. Breakdown products of the PFCs build up in the system because the body can’t excrete them fast enough, and that causes problems. You can’t keep somebody alive for months with just PFCs in their bloodstream.
What future medical uses of PFCs do you envision?
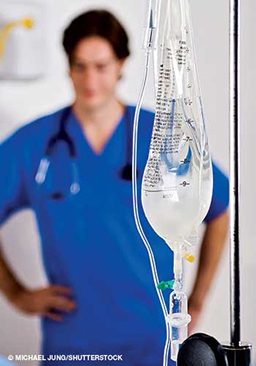
Hill: PFCs are effective in situations where you need short-term oxygen delivery without red blood cells. I don’t know if the military has looked at PFCs for use in trauma, but the compounds might be suitable for administration out in the field to enhance oxygen delivery during the evacuation to the hospital.
In cases of organs with transient ischemia (impaired or interrupted blood flow), PFCs might be able to get past the stricture because of the small size of PFC molecules relative to red blood cells. The PFCs could conduct oxygen delivery past a blocked artery until the vessel opened up again. In a patient with serious transient ischemic attacks (TIAs), for example, PFCs could preserve oxygenation of brain tissue. Doctors are starting to use angioplasty in the carotid artery and the artery inside the brain to try to open those. Perhaps potential strokes could be delayed or reduced in severity until those vessels could be reopened. A dose of PFCs administered to a patient with a pending stroke could increase oxygen delivery past the stenosed vessel and conceivably decrease the size of the stroke or reverse it altogether.
Bruce Spiess: Air embolism is nearly universal in heart surgery, and research with PFCs in the 1980s and ’90s showed up to a 98 percent reduction in bubble load. In war fighting, sudden death from improvised explosive devices (IEDs) is caused by rupture of alveoli (air sacs in the lungs) and subsequent air emboli leading to air bubbles in the heart and/or brain. This is analogous to pulmonary barotrauma in divers. Although death is often so sudden there is not time to administer PFCs before cardiac arrest, we believe PFCs could still be very useful in these circumstances.
How might PFCs be used to treat DCS?
Spiess: Today, we envision that PFCs would be used by first responders at the dive site and medical personnel in hospitals to treat DCS symptoms. The data consistently show that treatment with PFCs at the surface decreases mortality and, uniquely, seems to greatly reduce neurologic complications. (Research administering PFCs at depth was also conducted, but tremendous logistical difficulties limit the practicality of such an approach.)
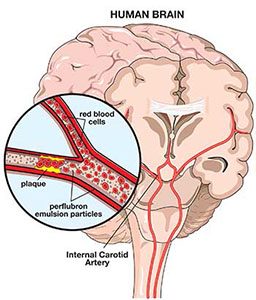
To achieve the greatest benefit from the PFCs, it is important that patients also breathe a high concentration of oxygen. There are several reasons for this. First, it takes advantage of the extra oxygen-carrying capacity of the PFCs, promoting oxygen delivery to tissues that might not be getting enough. Second, PFC particles are so small they can get to parts of the body where circulation is blocked by the air emboli of DCS, preventing red blood cells from getting in. Third, PFCs speed up the offloading of nitrogen and the shrinkage of bubbles. Finally, PFCs act as surfactants to speed up bubble dissolution and stop them from lodging in small blood vessels.
PFCs are administered as IV fluids in emulsions resembling milk. An emergency medical technician (EMT) could start an IV, administer PFCs, have the patient breathe 100 percent oxygen and transport to definitive care. Many sport-diving accidents occur in remote parts of the world where recompression chambers are not immediately available. We think starting treatment during evacuation could reduce complications and even be life saving. We also think using PFCs might improve safety of medical evacuations that require exposure to altitude during air transport.
What has the research taught us about these compounds?
Hill: We know what they can’t do, and we have a pretty good sense of what they can do. The right clinical scenario for their application is a transient problem that resolves soon. They’re novel compounds that do what they’re supposed to do, which is increase the oxygen dissolved in the fluid phase of the blood. The oxygen that gets to your cells has to be released from hemoglobin and red cells to diffuse through the fluid phase of the blood to get to the tissues. So increasing the solubility of oxygen in the fluid phase of the blood enhances tissue-oxygen delivery. Medicine hasn’t figured out the right way to use PFCs yet, but there will likely be an application for them eventually.
How has research and development progressed toward the use of PFCs for treating DCS?
Spiess: PFC research has made great progress. It has been about 30 years from the development of the first-generation compounds to today’s third- and fourth-generation compounds. Fluosol-DA 20% was approved by the Federal Drug Administration (FDA) and used in about 15,000 humans in the late 1980s and early 1990s. It was used as a treatment for acute blood loss and, to my knowledge, never used to treat acute DCS. That being said, I have used it experimentally for treating DCS and found it to be highly effective. Fluosol was eventually withdrawn from the market because it was cumbersome to use. It was supplied frozen, needed to be thawed and then sonicated (treated with ultrasound) to get it back into a usable emulsion. While 15,000 usages sounds like a good number, it is a failure if you are manufacturing and selling a drug, so it was withdrawn.
Today there are three or four compounds being investigated in the medical communities of the United States and other Western countries: Oxycyte (Oxygen Biotherapeutics Inc., North Carolina), Oxygent (New Alliance Pharmaceuticals/Sanguine Corporation, Georgia) and Perftoran (HemoTek Inc., Texas). Perftoran is available in Russia and perhaps other parts of the world, and Oxygent is being manufactured not only in the U.S. but in China, too, where it may already be available. In the U.S. we are working to respond to some final questions and concerns the FDA has regarding a side effect (possible toxicity related to platelet counts) of all PFC infusions. Once that side effect is better understood several human trials can move forward to the next round of research. I have proposed that a human trial in DCS (during transport and prior to recompression) would make great sense.
I believe we are three to five years away from having PFCs on the market for DCS indications. There is great science and support for these agents being first-line therapies in the future.
Meet the Experts
Steven E. Hill, M.D., is professor of anesthesiology and codirector of both the Acute Cardiothoracic Surgical Unit and the Duke Center for Blood Conservation at Duke University Medical Center. His research deals with clinical trials of blood-conservation techniques, oxygen therapeutics, thoracic analgesia and plasma volume expanders. He also studies research protocols involving oxygen therapeutic agents and the impact of blood management
on patient outcomes.
Bruce D. Spiess, M.D., FAHA, is former director and senior fellow at the Virginia Commonwealth University Reanimation Engineering Science Center and founder/director of the Flawless Operative Cardiovascular Unified Systems initiative to reduce human error in heart surgery. His work focuses primarily on oxygen therapeutics and blood substitutes, coagulation dysfunction with heart surgery and the risks of blood transfusion. With funding from the Office of Naval Research, Dr. Spiess is seeking ways to treat or prevent decompression sickness using perfluorocarbon emulsions.
© Alert Diver — Q3 Summer 2012