Gas density for divers
Humans have survived in relative comfort at depths up to 2,271 feet, breathing gas more than 12 times the density of surface air, but despite their ability to function in extreme conditions, minor differences among gas selections can make or break dives at any depth. For decades divers have found ways to mitigate the effects of pressure on the body without understanding them, but new research helps to explain human performance in challenging environments and provide evidence-based practices to minimize our risk in the water.
Gas density is one of the unknown mechanisms on the forefront of hyperbaric research. A recent paper1 by Gavin Anthony and Simon Mitchell brings together academic research and diving with a new understanding of gas requirements and how gas density can put divers at risk or help keep them safe.
Effects of Gas Density
Gas density is a simple concept with a complex but high-yield solution for two factors divers face: work of breathing (WOB) and carbon dioxide (CO2) elimination. Gas density is a measure of mass per unit volume in grams per liter (g/L), while WOB is an integral of pressure as a function of volume typically measured in kilopascals (kPa) or joules per liter (j/L). High WOB means it takes more effort to take a breath, and increasing gas density means it takes more effort to move that gas. An increase in WOB results in increased CO2 production and a decreased ability to inhale fresh gas, which compounds the second issue: CO2 production and retention. High partial pressures of arterial CO2 (PaCO2) can cause narcosis, hypercapnia and loss of consciousness. Exertion (or mechanical failure in a rebreather) can also cause these conditions, but gas density affects a second mechanism that exacerbates the situation.
The mechanism that removes CO2 from the blood functions by a pressure gradient between the partial pressure of inhaled CO2 (PiCO2) and PaCO2. PaCO2 is typically two magnitudes larger than PiCO2 (5.2 x 10-2 atmospheres absolute of pressure (ATA) vs. ~ 3.9 x 10-4 ATA), allowing for rapid diffusion of CO2 from the blood into the pulmonary filter and out of the body. Increasing PiCO2 via increased gas density decreases this gradient and reduces the ability of the body to eliminate CO2, further exacerbating symptoms of CO2 retention.
Doing Work
Equipment, respiratory rate and many other factors can affect WOB. Equipment differences are typically small, and divers tend to manage other relevant factors before they enter the water, leaving divers to contend with gas density as a primary modifier of WOB in many situations and a focal point for hyperbaric researchers. These experts are increasingly finding that the effects of depth are more extensive than previously understood, and many are suggesting it may be time to reconsider how and what we choose to breathe.
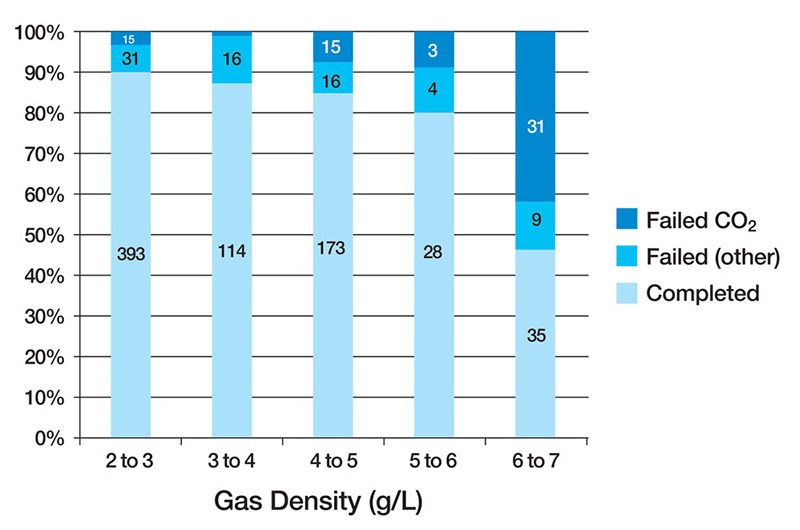
Anthony and Mitchell found that both rebreather and open-circuit divers retained a dangerous level of CO2 when their respired gas density reached 6.0g/L (normal air is approximately 1.293 g/L at 1 ATA) (Figure 1, Table 1). Previous studies2 found that CO2 retention during exercise at 6.8 ATA (8.79 g/L) resulted in two subjects requiring rescue from a wet pot (a hyperbaric chamber filled with water and pressurized to simulate a particular depth) due to CO2-induced incapacitation without any self-awareness of the symptoms. Further complicating the issue, Christian Lambertsen and his colleagues found that at just 4 ATA — the pressure we would experience at 99 feet of seawater (fsw), where the density of air is about 5.17 g/L — maximum expiratory gas flow and ventilation rate were nearly half of what they were at 1 ATA.3 The evidence appears to indicate that gas density has a profound effect on not just WOB and CO2 evolution and elimination but also our ability to effectively respire and exchange gas.
Anthony and Mitchell summarize their research by recommending an ideal gas density of 5.2 g/L, with an absolute maximum of 6.2 g/L. These numbers correspond with air diving at 102 fsw and 128 fsw, respectively.
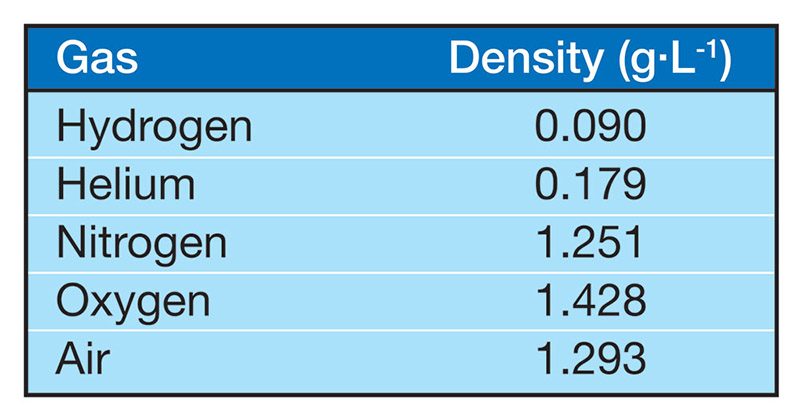
These limits coincide with recreational limits and significantly decrease the maximum operating depth (MOD) of many common gases. Enriched-air nitrox with 32 percent oxygen (EANx 32) at 110 fsw and 130 fsw results in densities of 5.6 g/L and 6.54 g/L, respectively, surpassing both recommended and hard limits. Common technical gases such as trimix 18/35 (18 percent oxygen, 35 percent helium and the balance nitrogen) and trimix 10/70 (10 percent oxygen and 70 percent helium) experience the same restrictions. Trimix 18/35 reaches a full 6.0 g/L at just 230 fsw (with a typical MOD of 300 fsw), and trimix 10/70 reaches a notable 10.29 g/L at its MOD of 495 fsw, far exceeding maximum recommendations and the 8.79 g/L mark that required divers to be rescued from a wet pot in the study by Dan Warkander and colleagues.2
Helium appears to be the immediate solution for divers who are concerned about WOB and correlated medical issues (immersion pulmonary edema, CO2 retention, etc.) and for technical divers who are doing even normal dives to moderate depths. Seven times less dense than nitrogen, helium also ameliorates the severity of narcosis but brings with it a host of new obstacles, including extended decompression, cost and high-pressure neurological syndrome (HPNS) concerns.
Our next steps depend heavily on the ongoing work of our hyperbaric community: In another decade we could have equipment that makes gas density a nonissue. For now we’re left to carefully mix our gases and learn all we can about human performance under pressure.
References
- Anthony G, Mitchell S. Respiratory physiology of rebreather diving. In: Pollock NW, Sellers SH, Godfrey JM, eds. Rebreathers and Scientific Diving. Proceedings of NPS/NOAA/DAN/AAUS June 16-19, 2015, Workshop. Durham, NC; 2016; 66-79.
- Warkander DE, Norfleet WT, Nagasawa GK, Lundgren CEG. CO2 retention with minimal symptoms but severe dysfunction during wet simulated dives to 6.8 ATA abs. Undersea Biomed. Res. 1990; 17(6):515-523.
- Lambertsen CJ, Gelfand R, Lever MJ, Bodammer G, Takano N, Reed TA, et al. Respiration and gas exchange during a 14-day continuous exposure to 5.2% O2 in N2 at pressure equivalent to 100 FSW (4 ATA). Aerosp. Med. 1973; 44:844-849.
- Doolette DJ, Mitchell SJ. Hyperbaric conditions. Comprehensive Physiol. 2011; 1:163-201.