Medical advances in recent decades have led to targeted treatments for some cancers. This has raised hopes for similar breakthroughs in the fights against other diseases. Politicians are getting involved, calling for further work in “precision medicine” and individualized approaches to patients. Medicine in the 20th century identified specific causes and treatments for many diseases, but treatment outcomes remain highly variable. We have learned that most diseases and injuries are caused by multiple factors, meaning that a specific event, condition or individual characteristic by itself is not generally sufficient to produce the disease. When our knowledge of any of the integral factors of a given disease is incomplete, we remain puzzled by varying outcomes, and our preventive and treatment interventions lack desirable precision and efficacy.
Sometimes unbeknownst to us there are several different sets of causal mechanisms that can lead to a specific disease. Other times individuals’ responses to a known set of causal factors remain variable for unknown reasons. Advances in genomic studies open new possibilities for identifying causes of variability — both in responses to harmful factors and in the efficacy of specific treatments. This has advanced modern oncology, and a similar approach could benefit other areas of medicine, too. First we will examine how genetic testing enables precision medicine in two common cancers, breast cancer and lung cancer, then we will discuss how precision medicine pertains to dive medicine.
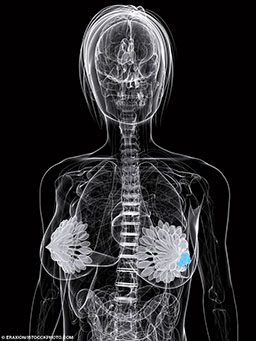
Breast cancer provides a good example of how genetic testing contributes to precision medicine. In the general population, breast cancer is caused by multiple factors. About 5 to 10 percent of breast cancer is caused by inherited genetic mutations. The two most important mutations related to breast cancer, BRCA1 and BRCA2, are present in 0.1-0.2 percent of the general population and in a much higher percentage in some minorities. The overall risk to women in the general population of developing cancer by age 70 is 12 percent. However, the average risk is much higher for carriers of BRCA1 (55-65 percent) and BRCA2 (45 percent).
While not all women with hereditary risk factors develop breast cancer, the risk for some women may be greater due to other known or unknown factors. Breast cancer is less common in men (0.1 percent), but in men who have the BRCA2 mutation the risk of breast cancer is about 7 percent — 70 times that of noncarriers. In addition to genetic testing of the individual, which provides information about the risk of acquiring the breast cancer, genetic testing of the cancer helps medical professionals establish a prognosis, choose effective therapies and avoid therapies to which the cancer is resistant.
In lung cancer today, genetic testing is unfortunately of less benefit, despite much work. Lung cancer is the leading cause of cancer death in men and women, and an external factor, cigarette smoking, is its number one cause — 90 percent of lung cancer is associated with cigarette smoking. However, only 10-15 percent of smokers develop lung cancer in their lifetime, suggesting that there may be a host of differences in susceptibility. A family history of lung cancer doubles the risk of developing lung cancer, but a specific inherited factor has not been identified. Genetic studies of lung cancers continue, not because we want to learn who can smoke risk-free (smoking causes many other cancers and serious diseases) but to find possible genetic drivers of cancer growth that could be targeted by therapy. At present, genetic testing may help guide the therapy in a small fraction of lung cancer cases. The most significant target for the prevention of lung cancer, however, is cessation of smoking, and preventive efforts need not wait for advances in medicine to eliminate 90 percent of lung cancer cases.
Problems with Precision in DCS
In decompression sickness (DCS), the magnitude and duration of exposure to pressure, the rate of decompression and some external factors determine the outcome of a dive. The role of tissue supersaturation with inert gas is notorious. The deeper and longer the dive and the faster the decompression, the greater the likelihood of supersaturation during ascent and of venous gas emboli (VGE), popularly called bubbles, which may result in DCS. In severe decompression accidents the consequences are generally grave without much variation among individuals due to the overwhelming significance of the external factors. However, in relatively mild dive exposures when the pressure and pressure change are limited, occurrence of VGE is variable but common, while occurrence of DCS is very rare but never zero.
The variability in both of these outcomes still escapes our comprehension. After the same dive, some divers may develop a lot of VGE, while others will not develop bubbles at all. In most divers who develop bubbles, the VGE are filtered out by the lungs and cause no harm. In a fraction of divers, however, VGE will pass through the right-to-left shunt from the veins into the arterial circulation, potentially blocking terminal arteries, damaging organs and causing symptoms of DCS. Even when this arterialization of bubbles occurs, few affected divers develop DCS. The only constant is that with more severe dive profiles (greater pressure, longer exposure duration and faster decompression) the average probability of DCS increases.
Contemporary dive-computer models measure exposure to an external factor — pressure — over time, but they cannot measure internal factors that modify the saturation and desaturation of the body. These include the amount of blood that perfuses tissues per unit of time, percent and distribution of body fat and other known and unknown factors. Algorithms used in dive computers take into account just the dive profile (the depth, time, breathing gas and rate of pressure change), assume an average body build and metabolic state, and predict the probability of DCS. However, it is clear that the risk is not the same for all individuals, but specific individual factors are not known. Figure 1 shows three stages of individually differentiated risk.
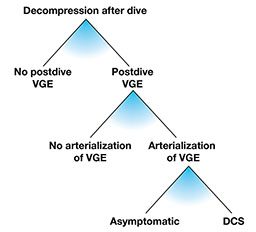
At each of these three stages there are factors that modify the effects and outcomes of exposure. In the absence of specific knowledge, the most common preventive strategy is to reduce supersaturation by limiting the depth/time exposure and ascent rate for all divers to avoid substantial bubbling — even though bubbles put only some divers (those with a right-to-left shunt [RLS]) at risk. Without bubbles (or with only a few), there will be no arterialization, even in divers with RLS, and there will be no DCS in those susceptible to arterial embolization.
Several approaches to mitigating postdive bubble occurrence have been studied; these include predive removal of hypothetical bubble nuclei by whole-body vibration, attempts to influence oxygen radicals or nitric oxide suspected of contributing to bubble generation, stimulation of heat-shock protein production and various other methods, including predive chocolate treats. Although some of these factors may reduce the amount of bubbles, the effects vary and may be of less importance than the individual variation in response to decompression.
If every diver had a consistent individual propensity for VGE production and could be classified as a “bubbler” or “nonbubbler” across a broad spectrum of reasonable dive exposures, safe exposure limits could be tailored individually. We would achieve greater precision if we could identify those divers who have persistent or occasional RLS and then customize a dive exposure for them. At present we already know that a large patent foramen ovale (PFO) enables arterialization and apparently increases the risk of DCS. A test for PFO is available. We can identify divers with a large PFO and close it, but this does not solve the problem for all divers because RLS may occur in lungs regardless of PFO. Risk of DCS due to pulmonary RLS may be more difficult to tackle because pulmonary RLS seems to be part of the normal physiological response to exercise and far more prevalent in the population than large PFOs.
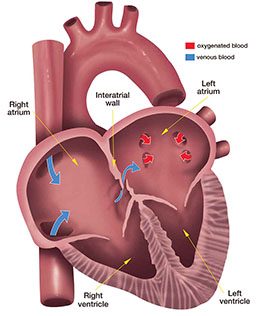
It is important to note that even if we could prevent the occurrence of VGE, DCS could still occur. VGE do not play a role in pain-only DCS. Some cases of spinal DCS may be caused by bubbles occurring locally in tissue, without bubbles in venous blood. Similarly, some cases of inner-ear DCS may be caused by local bubbles rather than by arterialized gas emboli. Cutaneous (skin) manifestations of DCS may be caused by various mechanisms, some involving embolization of arterialized VGE and others independent of VGE.
As in lung cancer, the definitive cause of DCS is an external physical factor that, unlike smoking, we cannot eliminate if we want to dive. But by controlling the magnitude of exposure, we can minimize the risk of DCS. There is still a lot of room to improve the precision of DCS prediction and develop individually tailored preventive exposure restrictions. This can be achieved by advancing VGE study methods and dive population studies to identify individuals who may easily produce VGE or are prone to arterialization.
Understanding why some divers easily produce VGE and why some are more prone to DCS in the case of VGE arterialization, however, will need a novel approach. One promising direction is the study of the innate immune system and inflammation, both of which are involved in the human body’s response to stress. Diving involves exposure to environmental changes that challenge the entire body and elicit immune responses and inflammation controlled by genetic mechanisms. However, measurable changes are rarely present in asymptomatic divers, making it difficult to study the transition of physiological adaptive stress responses into maladaptive or pathological reactions that lead to impaired organ function.
Genetic Studies
The idea that DCS, like breast cancer, may be promoted by a particular genetic or epigenetic variation attracts more and more researchers.
Genes are the basic physical units of inheritance passed from parents to offspring; they contain the information needed to specify traits. Humans have approximately 20,000 genes, an impressive collection that constitutes an individual’s genotype. Genes are grouped into 23 pairs of chromosomes, each pair containing approximately 3.1 billion distinct code elements. The information encoded in genes is expressed through the creation of proteins that build body structures and control body functions — the individual’s observable traits, called the phenotype.

This expression involves transcription of the code from genes to messenger molecules that leave the cell nuclei and enter the cell body, where they direct synthesis of a specific protein. Gene expression is affected by another layer of molecules (the epigenome), which varies from cell to cell. The relationship between genotype and phenotype is complicated further by gene interaction with the environment. Thus, studying genetics is complex and requires a lot of resources. So far, genetic studies in diving have just scratched the surface.
Changes in gene expression and transcription have been found in animals exposed to breath-hold and compressed-gas diving. Underwater diving exposes divers to hyperoxia and increased production of reactive oxygen species, which are involved with nearly every physiological process in cells, including programmed cell death (apoptosis). Response to this stress alone can alter gene expression and transcription. Similar stress may be caused by exercise in nondivers and by many other factors.
Some studies found that experienced divers, as compared with nondivers, showed persistent changes in pathways of apoptosis, inflammation and immune responses in blood transcriptomes, indicating a cellular state of sustained alertness toward exogenous stress. There were no measurable physiological differences, however, between the divers and nondivers. Changes found after scuba diving were typical of sublethal oxidative stress; they included suppression of lymphocyte activity and activation of the innate immune system. It was not possible to distinguish the effects of oxidative stress and of gas microbubbles. Changes were similar after additional dives, and they returned to normal in between the dives.
Whether there will be a dive analog of BRCA1 and BRCA2 is impossible to predict at this stage. But these are just the first steps in a long journey, and accelerating development in medicine and technology leaves little doubt that precision medicine for divers will be achieved.
© Alert Diver — Q1 Winter 2016