Virginie Papadopoulou, Ph.D., is a diver, researcher, former Marie Curie Fellow and current R.W. Hamilton Grant recipient who is quickly leaving her mark on a century-old field. With a background in theoretical physics and gas physiology and a doctorate in bioengineering, Papadopoulou is perfectly at home in the world of academia. It was a love of diving, however, that drove the native of Brussels, Belgium, to become one of the world’s leading researchers in the field of bubble science.
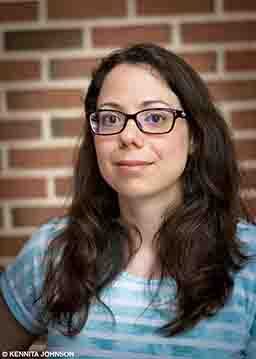
At age 10 Papadopoulou was obsessed with mystery novels and delighted in discovering the unknown through critical thinking and hard work. One of her favorite novels involved a thrilling adventure in an underwater cave, and upon reading it she knew that she wanted to become a diver. At 13 she convinced her father to enroll her and her family in a Discover Scuba course while on vacation at the Red Sea, and she began to drag the family along on yearly diving adventures.
As her experience in the water increased, so did her fascination with the science of dive medicine. When Papadopoulou took a divemaster course through her undergraduate program, she discovered that the decompression science covered in the course held her attention better than the theoretical physics she was studying for her degree. The distractions from her primary field of study compounded when a medical imaging course introduced her to ultrasound imaging technology, and she quickly shifted the focus of her education and work toward decompression research and echocardiography in diving medicine.
This research brought her to the lab of Mengxing Tang, Ph.D., at Imperial College London, where in short order she became an accomplished and extensively published researcher. Concurrently, she was part of PHYPODE — a European Commission-funded project of the Marie Curie Initial Training Networks (ITN) initiative — where she worked under the supervision of Costantino Balestra in Belgium and effectively got a crash course in human diving physiology. She also spent time in Greece collaborating with Thodoris Karapantsios’ laboratory in Thessaloniki, Greece, building a controlled system to record and model real-time bubble formation from decompression on different tissue surfaces.
Papadopoulou’s work has covered the breadth of the diving industry and spread into the fields of health care and disease treatment. Its focus, however, has always been on bubble mechanics. Her published literature covers topics and collaborations ranging from evaluating decompression stress with bubble counts to heart rate variability in skydivers to ultrasound-assisted delivery of drugs to the brain. She is fluent in five languages; an active dive instructor; a member of nearly a dozen professional hyperbaric, space medicine and engineering professional agencies; and has been recognized for her work by the American Association for the Advancement of Science (AAAS), the European Underwater and Baromedical Society (EUBS), the University of North Carolina, Imperial College London and others.
Currently a research assistant professor in the lab of Paul A. Dayton, Ph.D., at the University of North Carolina at Chapel Hill, Papadopoulou focuses her research on ultrasound microbubble cancer angiogenesis imaging and radiotherapy sensitization, both of which leverage her expertise in bubble modeling and imaging to create innovative medical therapies for some of the most difficult diseases to diagnose and treat. She also collaborates with the medical device startup SonoVol, cofounded by Dayton, whose mission is to create cutting-edge imaging solutions for the medical field. Their commercial system is the first ultrasound imaging device that can assess microvascular structure through acoustic angiography, an imaging technique enabled by microbbubble contrast agents.
Lately Papadopoulou has been working to foster multidisciplinary collaborations between biomedical and physiology researchers. The goal is improved understanding of how the body reacts to and recovers from external stressors and how to most accurately measure those changes. We appreciate her taking the time to speak with us.
You said diving brought you to the work you are doing now, but your research has covered an enormous range of topics. What ties together all your interests?
My primary interest in all my research has been bubble modeling. Reading decompression research, I am struck not just by what we know but also by what we do not know. There are almost more unknowns in the field than knowns, and this topic of study is almost a century old. Understanding how bubbles form in tissues before they become visible in the blood, how they behave and how our bodies react to them are the next steps in understanding how the human body handles gases both in diving and in medical scenarios.
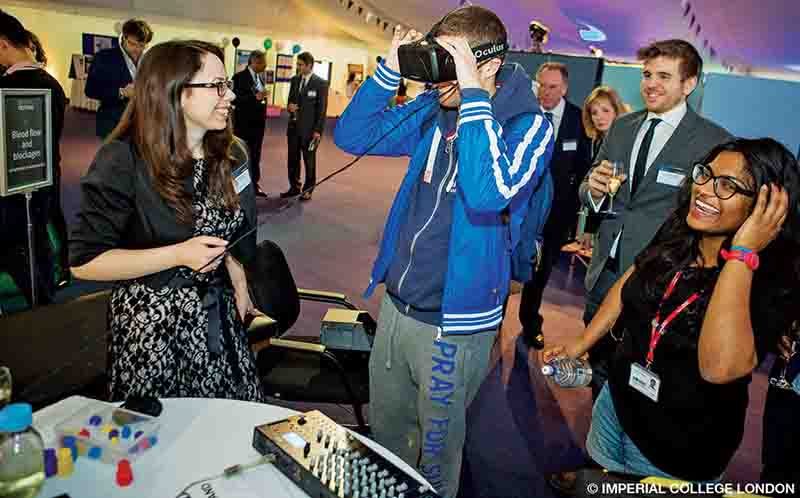
In the context of scuba diving, we aim to limit bubble growth during decompression, as higher bubble loads are known to increase the chance of decompression sickness (DCS). In medicine, however, very small, engineered microbubbles (micron-sized bubbles that contain perfluorocarbon gas and are typically encapsulated by a lipid shell) are injected in the bloodstream to enhance the signal from blood on ultrasound imaging. This allows us to image the vasculature and produce some incredibly detailed images without the potential costs or hazards of other currently available medical technologies.
We know those medical technologies are a focus of your current research. Where do we stand with cutting-edge bubble research being used in medical therapies?
Much of my current research involves using ultrasound technology and these microbubble contrast agents for new medical imaging and therapy applications. Our understanding of bubbles has allowed us to manipulate both the size and the structure of bubbles and to use them to create contrast in the body that can be viewed with ultrasound. By creating a stable nanobubble or microbubble with a specific protein on it that, for example, bonds with tumor cells, we can surround a tumor with those bubbles, view those with a diagnostic ultrasound and effectively image the tumor. This is particularly useful in patients with conditions such as renal failure that are contraindications for MRI and CT contrast imaging. Ultrasound imaging is nonionizing and has an extremely low risk of complications in the diagnostic imaging range. A few people may have allergic reactions to the bubble contrast, but that number is a very small percentage, and unlike in other types of imaging technologies, the body has little or no residual contrast to contend with after the procedure.
Individualized medicine shows a lot of promise for the near future, and the incredible understanding and control over bubbles that researchers and doctors now have may surprise many people. What do we know about the increased incidence of naturally occurring bubbles in certain divers? Are some divers predisposed to bubbling, and if so, are they at a greater risk of DCS?
It is hard to say whether some individuals are more likely to bubble than others. We observe using traditional B-mode (brightness) imaging that some people have more bubbles postdive than others, even for the same dive exposure. It is not clear, however, how much individual factors or dive conditions contribute to this, so isolating the contributions from hydration, ascent rate, water temperature, exercise, body composition, etc. is difficult. I also think we need to find a better way to measure bubble load before we can determine individual propensity to bubble, including a more continuous measurement postdive, additional information about bubble size (which is currently not assessed) and the ability to quantify tissue bubble formation directly, not just in vessels. This perception of some people bubbling more than others may in fact be a function of us not seeing the whole picture of decompression stress; factors we cannot currently measure, such as smaller microbubbles or tissue bubbles, may play a role.
What more can you tell us about the variability of DCS and the reasons that bubble grades might not be the best way to quantify DCS risk?
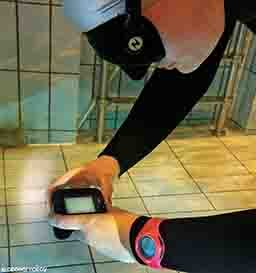
Researchers do B-mode, or Doppler, sonography to identify bubbles in divers. This type of imaging uses high-frequency sound waves to measure blood flow, and we can use it to observe bubbles moving in the blood of divers. Trained experts then give these a severity grade. We know that higher bubble grades correlate with a higher risk of DCS, but there is not a one-to-one relationship, and some people with very high grades are completely asymptomatic, whereas others with low grades get DCS. The way the body reacts to the bubbles is also important, so bubbles are not the whole picture; we want a more holistic picture of decompression stress. In addition, this mismatch between grades and DCS may be because of limitations in the way we are imaging, which is where my research into microbubbles comes into play. I have helped Peter Germonpré from Belgium assess a new counting method for analyzing B-mode recordings that may be more precise, and part of my Ph.D. work dealt with using computers to automate this counting to save on analysis time.
One limitation of B-mode is that it can only see large bubbles, those larger than 30-40 micrometers. One study I did had seven divers who all dived the same profile and then underwent bubble counts and a new contrast imaging technique inspired by the medical field. After their dives, some had big bubbles, and others did not, but using the new method of assessing microbubbles in the range of 1-10 micrometers, we observed an increase in signal in all seven subjects, which means that even divers with no large bubbles have small microbubbles postdive. The current theory is that there are many small bubbles like these that accompany larger bubbles, and there are many more small bubbles still in tissues that we could theoretically find. Measuring these small bubbles may be a way to better assess both decompression stress and DCS risk.
What would that mean for divers and the way we calculate DCS risk now?

Our current models use a binary assessment with only DCS and no DCS as outcomes, and there is no measure of changes in stress markers such as bubble load, oxidative stress and other physiological changes associated with diving that amount to decompression stress. I am working on approaching DCS from the other angle — I hope to better understand diving physiology by focusing on decompression stress and the ways it affects the human body. This mechanistic view of DCS creates a continuum of decompression stress, something that is much closer to the way we predict decompression effects in the body and devise personalized prevention strategies.
How close are we to observing microbubbles and accurately measuring our decompression risk?
This is the focus of some of the work I am preparing to do. I would like to bring the imaging techniques and technology I have taken from my work in Dr. Tang’s and Dr. Dayton’s labs and use them to create a new imaging device to view these bubbles and better understand the continuum of decompression stress. I have done similar things in the past: In Dr. Tang’s lab I used available technology and new acquisition strategies to combine two-dimensional flow information with ultrasound views to create a new and affordable way to measure blood flow with high 3-D accuracy in vessels. That measurement of blood velocity is incredibly important to identifying the progression of atherosclerosis, which is the leading cause of heart disease and related deaths. This method is more reliable and cheaper than other alternatives, and it has the potential to do a lot of good in medical applications.

And you would like to do something similar in the field of bubble imaging?
Yes. It is my hope that we can make an extremely precise tool to effectively measure bubbles that are not currently being imaged. I would like to look at how preconditioning minimizes bubbles. Astronauts prebreathe oxygen for several hours before they decompress in space outside their habitat. This minimizes pre-spacewalk nitrogen load. We do not know for sure, though, whether this is the most effective way to prepare them. If we understand exactly where and how bubbles form, we could come up with better ways to prevent DCS. With a tool that more accurately measures bubbles we will be able to really understand whether preconditioning with vibration, prebreathing or other techniques is truly effective in reducing DCS risk. There is absolutely value in approaching the issue of bubbling and DCS from both angles (the understanding and the treatment), but my work is focused on the understanding. I believe that the better we can understand the creation and behavior of bubbles, the better we can understand, prevent and treat DCS as well as other conditions that can benefit from the use of artificial bubbles.
Once we have a better understanding of how, when and why bubbles form, what do you think is next for dive medicine and medical applications of bubbles?
It is hard to know if you have a complete picture when you measure physiological changes after a dive. Researchers now are trying to come up with a controlled lab setup to quantify this microbubbling. The more we understand these microbubbles, the more we can be certain we have the whole picture with regard to DCS and decompression stress. If we do not know what really occurs between diving and DCS, we cannot identify risks, and we cannot effectively minimize our risk.
Explore More
© Alert Diver — Q1 2018 |