Richard Moon, M.D., has spent his career researching how the human body adapts to extreme environments. A professor of anesthesiology and pulmonary and critical care medicine at the Duke University School of Medicine and the medical director of the Center for Hyperbaric Medicine and Environmental Physiology at Duke University Medical Center, he has conducted cutting-edge research in hyperbaric medicine and diving science for nearly four decades. Moon’s work regarding the physiology of breathing has significantly advanced our understanding of the human body’s performance at extreme depths and in space.
After completing his medical education at McGill University Faculty of Medicine in Montreal, Canada, and serving several residencies, Moon had fellowships in biomedical engineering at the University of Toronto and in pulmonary medicine at Duke. He is well known for his hands-on approach to research, which has enhanced understanding about numerous difficult-to-study conditions. His career in diving medicine began with a record-setting study in 1981 that subjected three volunteers to a simulated dive to 2,250 feet for 43 days. The dive, part of the Atlantis III experiments, remains one of the deepest dives ever performed, and the study provided valuable information about the human body’s responses to extreme pressure and the limits of its adaptability.
Moon was one of the first researchers to identify the possible involvement of patent foramen ovale (PFO) in decompression sickness (DCS), and his case control study was the first published research on the subject. This work was a catalyst for more than a decade of research and led to general awareness among divers of the relationship of PFO and DCS.
At Duke, he has become an expert on the treatment of dive injuries such as DCS, arterial gas embolism (AGE) and immersion pulmonary edema (IPE) as well as on how gas under pressure affects divers’ physical and mental functions.
Moon, who recently traveled to the Himalayas to study how climbers adapt to extreme altitude, is also working with the U.S. Navy to study and prevent rebreather diver fatalities. We appreciate his willingness to discuss his work with us.
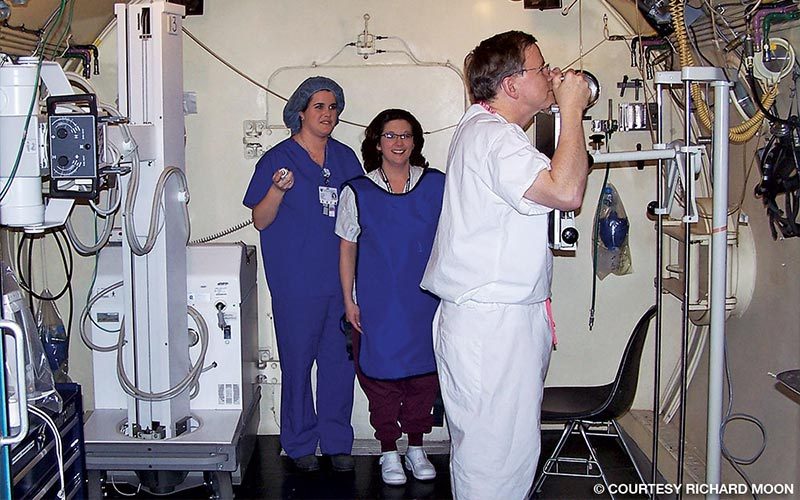
You’ve spent your career in a very specialized field. What led you to this kind of research?
My driving interest in work has always been the treatment and characterization of DCS. As a youth I became fascinated with the physiology of diving. Sea Hunt and Jacques Cousteau were obvious influences, and the works of Arthur C. Clarke and Edward Ellsberg fascinated me. After finishing my education at McGill University, I came to the U.S. to study diving physiology. I had trained as an internist in Canada and was training as a pulmonologist in the U.S. when my mentor, Enrico Camporesi, convinced me to become an anesthesiologist like him. He said becoming an anesthesiologist would make me a better pulmonologist, and I thought, It’s only two more years, so why not? I ended up liking anesthesiology and stuck with it. My day job is putting people to sleep (and waking them up again). As a pulmonologist and an anesthesiologist, I have spent a great deal of time studying the relationships between respiration and the human body; both fields are very relevant to my work in diving medicine.
Your diving medicine research began with the Atlantis experiments, which set pressure-exposure records that still stand today. What can you tell us about that work?
When I joined the team at the Duke Center for Hyperbaric Medicine and Environmental Physiology, I latched onto a group that was doing a lot of deep-diving research. As a pulmonologist with an interest in the mechanisms of gas exchange, I found the work fascinating. The Atlantis experiments were a series of four chamber dives with volunteer divers. We observed the effects of extreme pressure on the human body and determined what gases would be required to offset conditions such as high-pressure nervous syndrome (HPNS) that affect divers at great depths.
In the Atlantis experiments we used trimix — a combination of oxygen, helium and nitrogen, with around 5-10 percent nitrogen. The theory was that a narcotic gas such as nitrogen could offset the effects of depth and rate of compression in deep diving and attenuate the effects of HPNS. Nitrogen effectively attenuates the depth effect, but it is a very dense gas that does not resolve the issues associated with the necessary rate of compression. Its high density leads to pulmonary impairment at extreme depths. Other research teams at the time studied hydrogen as an alternative to nitrogen, but hydrogen poses some additional risks.
The data from these experiments are extremely useful for understanding human physiology and the effects of depth on the body, but with robots as good as they are, it is unlikely that humans will ever dive that deep again. Robots, for example, fixed the 5,000-feet-deep wellhead that created the BP oil spill in 2010. This is one of the reasons that our record still stands. Only one similar dive has exceeded our maximum simulated depth of 2,250 feet, and it is unlikely that will change in the near future.
Did you encounter any unexpected issues with extreme depth during the Atlantis experiments?
We got a lot of interesting data from our deep-diving research, but something that was perhaps unexpected was having someone become manic at depth in the chamber. During the Atlantis IV experiment, a volunteer developed agitation, hyperactivity, racing thoughts and insomnia at around 2,050 feet. Benzodiazepines successfully controlled these symptoms at that depth, but at 2,132 feet the symptoms returned and progressed into a manialike syndrome that lasted for two days. This prevented completion of the studies and necessitated early decompression.
There was a lot of deep-diving research going on in the 1980s in Germany, France, Japan and Norway, and most of the people running these experiments also had someone become manic during a dive.
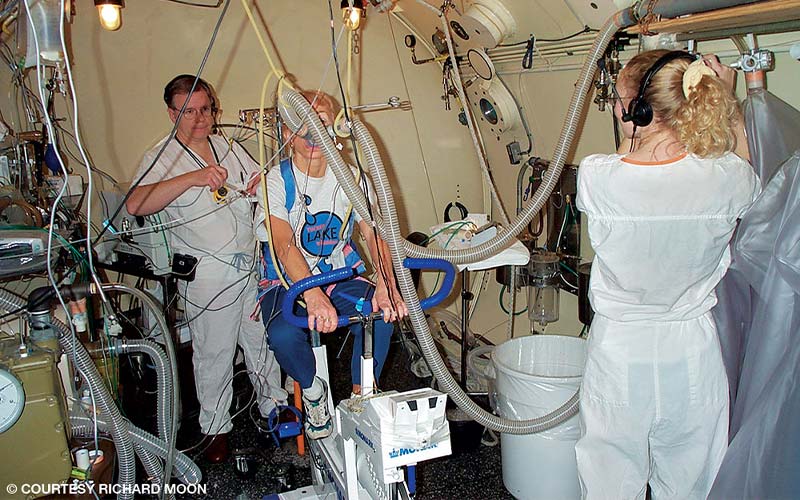
You were one of the first researchers to identify a relationship between PFO and DCS. There has been significant research on PFO closure since you made that discovery. Is there still work to be done on that front?
We discovered the link between PFO and DCS in the mid-1980s. The National Oceanic and Atmospheric Administration (NOAA) sponsored research that allowed us to bring back patients we had treated who were previously paraplegic because of DCS. This gave us insight into the effects of PFOs and demonstrated the substantial capabilities of hyperbaric oxygen therapy. These patients who had originally come to us with severe DCS and partial or complete paralysis were walking and functional.
As an aside, we learned a lot about managing spinal DCS from the initial treatment of these patients. Because they could not move their legs, their risk of pulmonary embolism was significantly increased. It wasn’t clear that traditional prophylactics would adequately attenuate the risk of pulmonary embolism, and we discovered that we had to scan the legs for clots to manage that risk.
It is fairly well established that a PFO is related to serious and repeated DCS. I think it is a closed issue at this point. In some individuals, closing a significant PFO will decrease the risk of DCS. Approximately one-third of people who get DCS, however, will have serious symptoms regardless of whether they have a PFO — the main risk factor for DCS is still the exposure (i.e., depth and time). The discussion that culminated in DAN’s 2015 Patent Foramen Ovale and Fitness to Dive Consensus Workshop answered most of our questions on the topic.
You have done a great deal of research on IPE, particularly in divers and other athletes. What prompted this work, and what have you found?
IPE first became a concern when it appeared in otherwise healthy Navy SEALs who had undergone rigorous physical exams and were in excellent shape. Several SEALs had problems with IPE while swimming in California’s cold San Diego Bay during training, and we worked with the Navy to research the risk factors and possible treatments. This was particularly interesting because these military patients had no obvious preconditions to IPE, such as high blood pressure or cardiac defects.
In our research we found that increased pulmonary artery pressure and wedge pressure were risk factors for IPE. We identified subjects with multiple cases of IPE and compared their hemodynamics. We used sildenafil (Viagra) and other drugs to attempt to prevent IPE. In one study we submerged patients with a history of IPE in 68°F water and then warmed them out of the water, gave them sildenafil and submerged them again. We found decreased wedge and pulmonary artery pressures after the administration of the drug, and one subject went so far as to have her results sent to her primary care physician. That physician prescribed prophylactic Viagra for her to use before triathlons, and she has had good results with that treatment.
Other research on the body’s reaction to cold water resulted in several people with unexplained symptoms such as an extreme increase in blood pressure upon submersion in cold water and an otherwise normal heart that expanded significantly beyond the norm and produced mitral regurgitation. We are always interested in looking at cases like these or unexplained cases of IPE; that is one of the diagnostic services we provide at Duke.
We still question why these at-risk individuals have increased pressure in their pulmonary arteries. We are currently using echocardiography on submerged subjects to research this condition. We are waiting to get back results now, but one theory we have is that individuals susceptible to IPE have slightly stiffer left ventricles, which could lead to higher filling pressure.
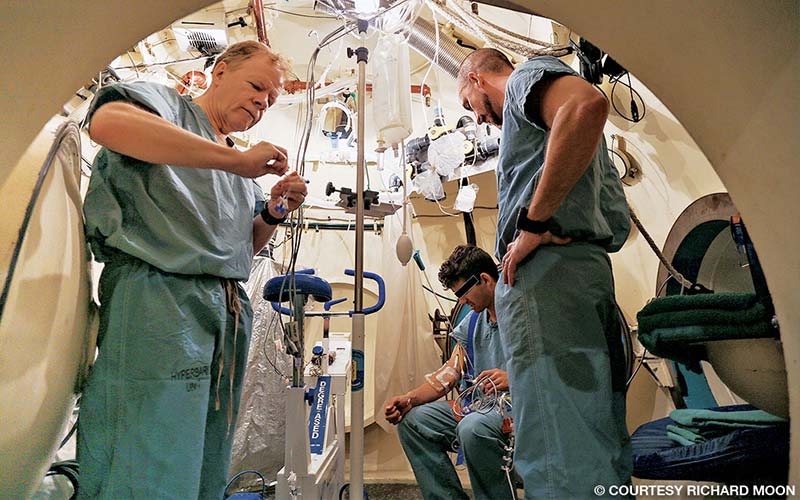
You have studied the human body’s limits in low-oxygen and high-altitude conditions. What have you learned from this research?
We have spent a lot of time working to prevent DCS in astronauts and studying the adaptations of climbers in high-altitude environments. I just returned from a field study that involved trekking more than 40 miles and ascending 8,300 feet to reach the Everest Base Camp at an altitude of 17,651 feet. The ambient pressure there is roughly half that of sea level, and we did some fascinating work measuring the oxygen saturation of the climbers preparing to attempt the summit. We found fully functional individuals walking around the camp with saturation levels as low as 60 percent. This would normally indicate a significant functional deficit, but their bodies had adapted to cope with the conditions.
You’ve recently been working to reduce the number of fatalities among rebreather divers. What causes most of these fatalities, and how are you addressing this issue?
Our work with rebreather divers led to a grant from the U.S. Navy to develop a monitoring device to prevent rebreather diver deaths. Rebreather diving is booming, and people enjoy using rebreathers recreationally for the lack of bubbles, reduced gas usage and decompression benefits. The most common cause of death in rebreather divers is hypoxia (oxygen deficiency), and we have shown that pulse oximetry can be used to provide a reasonable warning before hypoxia causes a loss of consciousness.
Our future research will focus on the use of pulse oximetry with rebreather diving, and we have proposed a design for a small forehead-mounted pulse oximeter that’s connected to a heads-up display. Pulse oximetry can give the diver a warning almost a minute before they become dangerously hypoxic — even during exercise. This device could make great strides in saving the lives of rebreather divers. The work is ongoing, but preliminary results have been very promising.
What insight have you gained from your research as a whole?
I find that the human body’s reactions in the most extreme conditions are quite relevant to our understanding of both clinical medicine and the human condition. Our experiments at depth and altitude have taught us a remarkable amount about the function and adaptability of the human body, particularly the lungs. Diving research, hypoxia research and hyperbaric research are all very applicable to our general understanding of the human body.
© Alert Diver — Q3 2017 |