Diving imposes significant challenges to the respiratory system. Lung conditions are among the most common reasons people fail diving medical examinations — and of course we depend on our lungs for survival. Thus it is important that divers know what limitations to pulmonary function occur while diving.
Gas Exchange
The lungs’ most important function is to facilitate gas exchange: supplying oxygen to the bloodstream and extracting and exhaling carbon dioxide (CO2). Of these two processes, the exhalation of CO2 is actually the main driver of breathing. The CO2 combines with the water in the blood to form carbonic acid (which dissociates as hydrogen and bicarbonate ions).1 The change in the pH of the blood is sensed by the brain and serves as the trigger to stimulate breathing. To maintain the pH balance in the blood, the lungs must be able to “blow off” the CO2 that is produced by the body.
To do so, a certain volume of air is used to dilute the CO2 in the alveoli of the lungs by means of inhalation and exhalation. A given amount of exercise also causes a corresponding amount of CO2 to be produced by the body, and the same amount of alveolar ventilation is required to eliminate the CO2 regardless of whether a person is at the surface or at depth. Divers’ bodies have to work harder to eliminate CO2 during dives and have a limited capacity to perform this work. The following eight factors are among the primary reasons for why this happens.
Breathing-Gas Density Increases with Depth
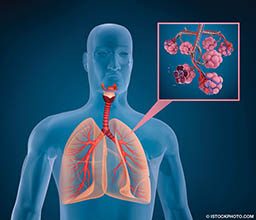
Divers’ breathing gas is compressed by the increased ambient pressure at depth. A volume of gas at depth contains more molecules than the same volume of gas at the surface. Increased density also increases the viscosity of the gas, which means that it is more difficult to breathe the same volume of gas at depth. One study indicated that at a depth of 100 feet, the maximum volume of gas that you can inhale and exhale in one minute (maximum voluntary ventilation) is approximately half of what it would be at the surface. Likewise, the volume of gas that you can forcefully exhale in one second is reduced by almost 10 percent at 33 feet and about 13 percent at 100 feet. The maximum flow rate that you can produce (peak expiratory flow rate) is reduced by about 23 percent at 33 feet and by 39 percent at 100 feet. These are significant limitations on divers’ respiratory systems.
Physiological Effects of Immersion
During immersion, blood that is normally distributed to the limbs moves into the body’s central space (i.e., the chest). About 700 ml of blood is shifted in this manner, and a large portion of it is accommodated by the blood vessels of the heart and lungs. This makes the lungs stiffer, meaning they have reduced elastic recoil and that breathing requires more effort than normal. The blood also takes up volume. This means that the total volume of the lungs would be reduced as a result of the fluid shifts. Wearing a tight-fitting wetsuit compounds this redistribution of fluid, shifting additional blood into the central space by compressing the blood vessels in the limbs. Further promoting this fluid shift is when diving occurs in a relatively cold environment, meaning that peripheral vasoconstriction also contributes to a central fluid shift.
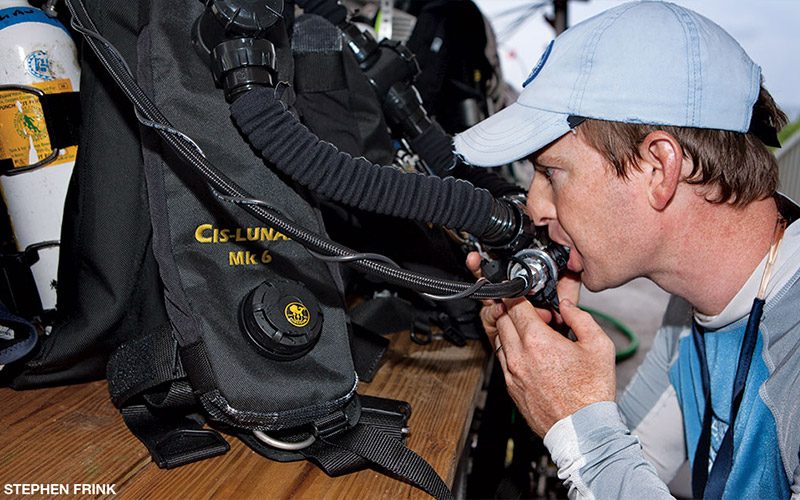
Altered Regulation of Breathing
Some studies have found that even if a diver did not perform at the maximum level of exercise during a dive and did not reach his or her ventilation limits, the CO2 levels in the diver’s blood would still be elevated. Common sense would dictate that the CO2 (and carbonic acid formed) would trigger the brain to increase the breathing rate, but this expected response was not seen. This would mean that even though maximum breathing levels were not exceeded, the brain “chose” to allow a higher level of CO2 in the body under conditions in which the work of breathing was increased. This demonstrates an alteration in the operation of the brain’s respiratory regulation center, which is not yet fully understood. At least four hypotheses have been proposed to explain this phenomenon: 1) the effects of an increased partial pressure of oxygen during diving, 2) certain effects of nitrogen narcosis, 3) a direct effect of the increased pressure and 4) changes in the way the brain controls ventilation when the workload on the lungs is increased. More research is required to understand the exact mechanism.
Increased “Dead Space” During Diving
Dead space refers to areas in which there is ventilation (i.e., gas movement), but no gas exchange takes place. Since gas exchange happens only in the alveoli, the conducting airways such as the trachea and bronchi are dead space. Diving equipment adds additional space, usually less than 200 ml. The dead space in a diving regulator is typically less than 100 ml. Nonetheless, considering that the volume of a normal breath is about 500 ml, dead space adds up quickly, leading to elevated breathing volumes. In addition to this physical dead space, there is physiological dead space that is also increased during diving. A full description of this is beyond the scope of this article, but suffice it to say that there are additional mechanisms by which ventilation in a diver is compromised.
Rebreathing
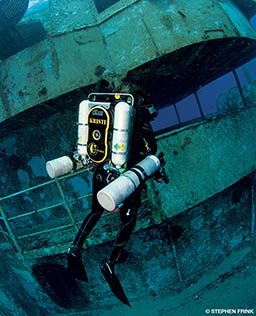
Rebreather divers should take particular care to prevent CO2 toxicity as a result of rebreathing gas. Hypercapnia (elevated CO2 in the blood) is probably the first consideration in the differential diagnosis of a diver who lost consciousness while diving on a rebreather (the other two most likely explanations are hypoxia [low oxygen] and hyperoxia [excess oxygen]). Rebreathing CO2 is possible if the scrubber material (soda lime) is not packed correctly and channeling occurs. Channeling is a phenomenon by which gas moves through channels in the scrubber material, which limits removal of CO2. To reduce the risk of rebreathing CO2, divers are taught to “prebreathe” the rebreather for five minutes before diving. A study published in 2015 demonstrated that this approach is not effective in detecting all scrubber failures, and some study participants even failed to recognize a problem when given prebreathing rebreathers that had no scrubber.2
If rebreathing occurs due to scrubber failure, hypercapnia will begin to stimulate the respiratory control center of the brain to increase the rate and depth of breathing. As hypercapnia continues to escalate, divers may experience severe breathlessness and may panic. In severe cases, hypercapnia can lead to loss of consciousness, which happens to rebreather divers from time to time. There is a concern that divers who are not sensitive to hypercapnia will experience only minor symptoms, such as shortness of breath, before suffering loss of consciousness. Even mild hypercapnia can have adverse implications, however, particularly for rebreather and nitrox divers: Elevated CO2 levels increase the risk for central nervous system (CNS) oxygen toxicity, which can cause convulsions underwater and drowning.
Poor Diving Procedures
A very rapid descent promotes a buildup of CO2 and is usually associated with a decreased minute volume (the amount of gas breathed in one minute), which can lead to hypercapnia. Another practice that can lead to a decreased minute volume is skip breathing — holding your breath for short periods between inhalation and exhalation. The intention of divers who do this is to preserve gas, but it leads to an increased level of CO2 (and is a bad idea for other reasons as well). This then leads to the symptoms described in the previous section.
Gas Contamination
Apart from the various physiological reasons hypercapnia may occur during a dive, contamination of the breathing-gas supply should also be considered. If the compressor intake is situated where contamination is possible, the diver may inhale CO2 at higher concentrations than in normal air. This would put the diver at a distinct disadvantage for obvious reasons. The concentration of CO2 in diving cylinders should not exceed 500 ppm, and operators are legally required to regularly test the air they supply to divers.

CO2 Retainers
Some people do not have a proportional response to hypercapnia (i.e., their breathing does not adjust fully to maintain baseline CO2 levels), and these individuals can have very high — even dangerous — levels of CO2 in their blood. These people are referred to as CO2 retainers. A number of studies have evaluated the standards to screen for such individuals during dive medical fitness evaluations. These studies concluded that there is currently no screening test that would enable us to identify these individuals. At best, the currently available tests would disqualify a large number of normal people by incorrectly identifying them as CO2 retainers. Screening is thus not advised.
Conclusion
Hypercapnia is hazardous while diving because of the risk of impairment or complete loss of consciousness. Milder, gradual buildup of CO2 may cause a throbbing headache or the feeling that the diver is not getting enough air. This may lead to a panicked response in which the diver bolts for the surface, potentially leading to decompression sickness or pulmonary barotrauma and arterial gas embolism if the diver doesn’t exhale sufficiently during the rapid ascent. Hypercapnia also increases the risk for decompression sickness, nitrogen narcosis and CNS oxygen toxicity mainly because of the increased blood perfusion caused by the CO2-induced dilation of blood vessels.
All divers are likely to have increased CO2 levels (to some degree) during a dive. The manifestations are usually minor — such as a throbbing headache — and if the diver realizes this and immediately resumes normal breathing, the headache may subside. If not, it is wise to shorten the dive but also to make sure that adequate ventilation is maintained during ascent. Relaxed, deep breathing at the safety stop also helps in many cases.
There are other causes of headache, of course, including carbon monoxide contamination, so diving-related headaches should not be dismissed as trivial. If in doubt, call DAN®. Be vigilant; maintain awareness of the signs of hypercapnia.
References
- Camporesi EM, Bosco G. Ventilation, gas exchange, and exercise under pressure. In: Brubakk AO, Neuman TS, eds. Bennett and Elliott’s Physiology and Medicine of Diving, 5th ed. Edinburgh, UK: Saunders; 2003.
- Deng C, Pollock NW, Gant N, Hannam JA, Dooley A, Mesley P, Mitchell SJ. 2015. The five-minute prebreathe in evaluating carbon dioxide absorption in a closed-circuit rebreather: a randomized single-blind study. Diving Hyperb Med 2015; 45(1):16-24.
- Doolette DJ, Mitchell SJ. Hyperbaric conditions. Compr Physiol 2011; 1(1):163-201.
© Alert Diver — Q2 2017 |